A new hypothesis to explain aging
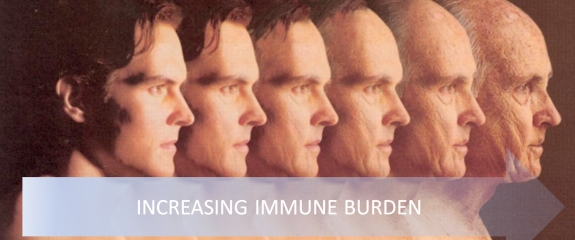
Introduction
Few topics are as simultaneously fascinating and mysterious as aging. All living creatures share the will to live and humans even try to combat aging investing countless efforts and resources in cosmetics, wellness programs and hypothetical anti-aging supplements. And yet, despite our continued interest and in the face of the general progress that science has made, our understanding of the aging process remains elusive.
After having sequenced the genomes of all relevant species for laboratory testing and beyond, the genomic era seems to have given way to a new one, epigenetics. It is a growing field that encompasses those mechanisms regulating gene expression that do not involve changes in the genotype. We are in a time where epigenetics has become fashionable. Since both aging and epigenetic modifications have time as a common factor, it is not surprising that many researchers have focused on finding a connection between these two phenomena. As a matter of fact, more than twenty scientific groups are currently studying the putative connection between aging and epigenetics, producing some 500 relevant publications. Evidence indeed suggests that epigenetic factors, including DNA methylation and histone modifications may affect the aging process. However, no one is yet able to describe a clear pathway through which genes may be sequentially switched on and off as aging progresses. Neither is there a ‘road map’ that can demonstrate how the cascade of epigenetic modifications alters our longevity nor any conclusive epigenetic biomarker suitable to track how we age. Perhaps the one solid lesson we have learned from the study of epigenetics is that epigenetic trends progressively diverge as we age. This single observation serves to help us understand – perhaps – why monozygotic twins are so alike in their youth and yet so different in maturity. Regarding a biological explanation of aging though, epigenetics falls clearly short.
A second, and perhaps more widely spread hypothesis of aging relates to telomere length and shortening. Telomeres are a region at the tips of our chromosomes (the structures containing our genes coded in DNA). Whenever a cell duplicates, it must first duplicate its chromosomes. But the DNA replication machinery has a constraint; it cannot duplicate the very tips of a DNA molecule. Thus, telomeres become progressively shorter with each cell division. So according to this hypothesis, cells undergo many divisions during our life up to the point where they end up with chromosomes bearing short telomeres. At some point telomeres become critically short and the cell will not undergo any further division (highlighting the necessity of telomeres). In this model, aging is often explained as a consequence of the gradual loss of cell replication capacity, which in turn means an eventual loss of organ function.
The beauty of this hypothesis resides in its simplicity. Unlike the complex multifactorial epigenetic model, there is just one single variable to explain aging: telomere length. Simplicity apart, there are also important observations that, while not giving direct support, are compatible with this model. For example, telomere shortening in normal somatic cells has been demonstrated in both in vitro and in vivo models. Moreover, there are rare disorders such as dyskeratosis congenita and aplastic anemia where cells have chromosomes with very short telomeres and the affected individuals have significantly shorter lifespans. And yet, however appealing the telomeres length hypothesis might be, it has a major drawback; telomeres are pretty much intact in length at death. In addition, setting aside pathological conditions, telomere length varies widely in humans without any clear impact on lifespan per se. This observation has been documented repeatedly in over a thousand publications and strongly suggests that a causal relation between telomere length and aging is unlikely.
An alternative means by which telomeres could be instrumental in the aging process would be through their shortening. In other words, the absolute telomere length is not important for aging but the rate of shortening could be; influencing aging through what it has been termed positional effect. Telomeres are highly compacted regions of the chromosomes. Through a process of cooperative forces the neighboring non-telomeric regions of the chromosome also experience a degree of compaction. This has an impact on gene expression. Any distal gene, one that is close to a telomere, will tend to be silenced due to this condensation because it is not accessible to the transcription factors. As telomeres shorten though, the compaction pressure relaxes, allowing previously silenced genes to be expressed. The positional effect has been reported in several species including humans but its impact on aging remains unknwon. As in the case of the epigenetic model, it might be expected to find a correlation between aging and a temporal pattern of newly activated genes. And, as it happens in the epigenetic model, no such hypothetical pattern has been observed. This leaves us with two possible alternatives: 1) telomere shortening is not involved in aging; 2) telomere shortening is related but distal genes shuffle, thus, confounding the scientific documentation of a gene expression cascade related to the aging phenomena. This second alternative seems feasible in view of the recent results obtained in the 1000 Genomes Project that is describing more variability in terms of gene location and presence than previously thought. If so, we would be concluding indirectly that telomere shortening is not a causal factor for aging as it would depend on the presence and the relative location of genes in the distal portion of the chromosomes. Therefore, it is reasonable to conclude that telomeres are not directly responsible for the aging process; neither in terms of length, nor in terms of rate of shortening.
How then do we explain a disease like dyskeratosis congenita? A detailed explanation is beyond the scope of this article. However, put simply, there is a second player in the telomere model. The enzyme telomerase serves to elongate telomeres and works to reverse the normal telomere shortening that results from cell duplication. Normally, this enzyme is only expressed in germ and stem cells. The introduction of telomerase makes the model more complicated for we know very little about its expression pattern as it raises new questions. For example, is it constantly expressed and functional in stem cells like those of the hematopoietic tissue or is its gene switched on and off cyclically? Does it only operate on critically short telomeres? Etc., etc. (Note that this ignorance clearly affects our perception of the ‘shortening rate’). The issue is that diseases like dyskeratosis congenita are characterized by mutations in the telomerase gene, which impair telomerase activity. With the addition of this new and poorly understood variable to the model it is tempting to give back some credit to telomere length as a key player in the process of aging. However, sticking to strict logical thinking, all we know is that the lack of telomerase is causing a disease. Short telomeres are the consequence of this lack but the enzyme might exert secondary functions not related to telomere elongation that are – directly or indirectly – the real cause of aging. As a matter of fact, non-telomeric telomerase functions have been described relating telomerase to gene expression, signaling pathways and mitochondrial function.
Truth to tell, the overall conclusion is uninspiring: we are still pretty ignorant about the factors that govern aging.
Proposed hypothesis
The first law of thermodynamics states that the total energy of the universe is constant. This law is actually a ‘principle’ because it remains unproved. We believe this law simply because all attempts to revoke it have failed. Similarly, the fact that all efforts to explain aging have been unsatisfactory makes me believe that aging is a complex multifactorial process.
Therefore, the hypothesis I am going to introduce does not intend to account for all about aging. It is introduced solely as a complementary point of view to enrich our vision on this phenomenon.
I start making the following observations:
- Aging is characterized by many features but two remarkable ones are inflammation and functioning of the immune system decline. By their nature, it seems reasonable to assume these two characteristics are most probably inter-related.
- The immune system protects us from infectious disease by recognizing and attacking structures (termed antigens) present in viruses and bacteria. The point I would like to make here is that these antigens are small molecules very similar to those composing our own cellular structures. Thus, the immune system has to learn to distinguish ‘self’ from ‘non-self’ antigens simply to fight against invading microorganisms but not against our own body. It is well known that this process of ‘education’ really exists and – here comes the important observation – although it continues throughout our lives, it is most active during fetal development.
- Throughout our lives, our cells are synthetizing proteins, which hang around executing their functions until they become degraded. This process occurs repeatedly in cycles and thus, the process is termed ‘protein turn-over’. But protein turn-over is not 100% effective. So cells will tend to accumulate denatured (i.e. not with the original and functional structure) and unfunctional proteins which escape degradation. Following on from my use of the first law of thermodynamics above, I will also refer to the second one here: that entropy (the measure of disorder) always increases in a closed system. Thus, the accumulation of unfunctional protein can be considered as a consequence of the second law in living organisms.
- To my knowledge, there is at least another mechanism to alter the standard conformation of proteins: non-enzymatic glycosylation. The sugar in our bloodstream and cells covalently binds to proteins that finds in its way. This process is notorious in poorly controlled diabetic people who prematurely suffer from different diseases related to aging. A big part of these non-canonical glycosylated proteins will be degraded. However, there will also be a residual fraction accumulating progressively as we become older.
My hypothesis combines these four observations into a single model: throughout our lives, our bodies increasingly accumulate a mass of non-standard proteins. These proteins are partially (or totally) denatured either by natural changes in conformation or by actions such as non-enzymatic glycosylation. These non-standard proteins become the source of new antigens for our immune system. As these molecules were scarce during fetal maturation, our immune system did not have the chance to learn that these antigens are also ‘self’. As I pointed out before, the immune system has the chance to learn during the whole life but in a much less efficient way in adulthood. Therefore, as we age there is an increasing opportunity to raise an immunological response against our own proteins. The immune system will start an incremental fight against our own bodies, attacking functional cells in the organism, producing inflammation, and finally exhausting itself to the point that can no longer fight against a real external threat.
This hypothesis needs to be tested and I leave the task to the reader who wants to work in science. But there is a number of observations that provides support to this idea:
- It is well known that glycosylated proteins can raise an immune response not started by the unglycosylated version of the same protein.
- Changes in the 3D protein structure can produce structural antibodies.
- Low-calorie diets have a positive effect on the lifespan of rodents. This is what one would expect if glycosylation were one of the problems of aging.
How could this hypothesis be tested? As I said before, I leave the answer to the curious reader. However, I can readily think of two experiments: a) if a low-calorie diet improves normal mice´s lifespan, and if this were related to the immune system, we should not see this effect in nude mice also subject to low-calorie nutrition. b) if either by a drug or over-expression of certain proteins one could improve the proteasome activity in an animal model, then this animal should live longer if the hypothesis were correct.
The idea that our main source of energy (sugar) and the action of the second law of thermodynamics condemn our lives is tantalizing. Moreover, and contrary to what is fashionable nowadays, genetics would play only a secondary modulating role in this scenario. I do not know whether the model will prove to be correct but – in any case – I hope it let other scientists to challenge it. After all, reality is always more complicated than we think.
About the Author:
Dr Marcelo Viegas studied Biology and Computer Sciences at the University of Buenos Aires, Argentina. After a short phase of work at a pharmaceutical company, he became a doctoral fellow in Prof. Andreas Kulozik and Prof. Matthias Hentze laboratory at the European Molecular Biology Laboratory (EMBL) where he studied the physiological implications of the Nonsense-mediated mRNA decay pathway. He was awarded a Ph.D. by EMBL and the University of Heidelberg in 2007. Thereafter, Dr. Viegas moved to the industry working for several biotechnology companies focused in molecular diagnostics and personalized medicine. He served as Technical Director at Abyntek, Director of Development at Transbiomed and Chief Scientific Officer at Abyntek and Life Length. It was in this last company where his concern about the aging process developed.